Current Research Projects and Research Directions
Uncertain Nonlinearities in Groundwater Flow Models
[in collaboration with Prof. Dr. M. Bachmayr & Mr. S. Boisserée (Applied Mathematics – RWTH Aachen)]
The fluid flow in porous rocks is governed by complex and non-linear Partial Differential Equations (PDEs). In this project we are investigating the solvability and the behavior of non-linear porous-flow equations. These equations describe the fluid flow within deforming rocks. In particular, the complex volumetric rheology of rocks is investigated. The results of this study have many implications for energy applications that include the integrity and the permeability evolution of reservoir rocks.
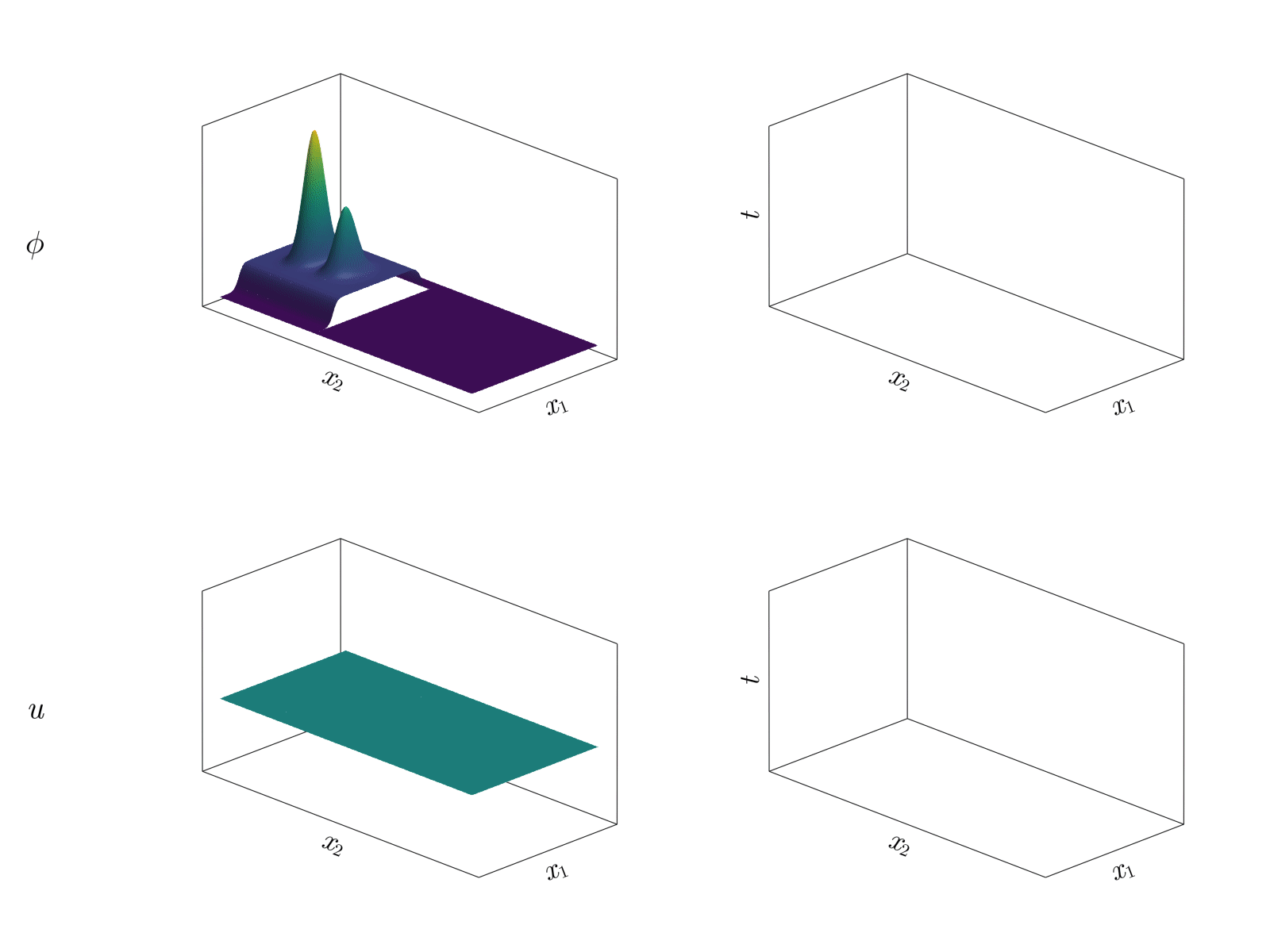
Porosity evolution (top row) and effective pressure evolution (bottom row) during compaction-driven fluid flow. The computational grid on the right spans the whole space-time domain. Figure courtesy of S. Boisserée.
Grain-scale pressure variations in metamorphic rocks
[in collaboration with Dr. M. Mazzucchelli (Uni. Lausanne), & Prof. Y. Podladchikov (Uni. Lausanne)]
Metamorphic rocks are always deformed when recrystallized. Even in the absence of far-field deformation, the volumetric thermoelastic strains can lead to the development of GPa level of stresses at the mineral scale. For this reason, we develop new mechanical models that allow the quantification of the deformation in composite materials such as rocks. Such models are tested using spectroscopic techniques (e.g. Raman spectroscopy) and can provide insightful information regarding the development of residual stresses.
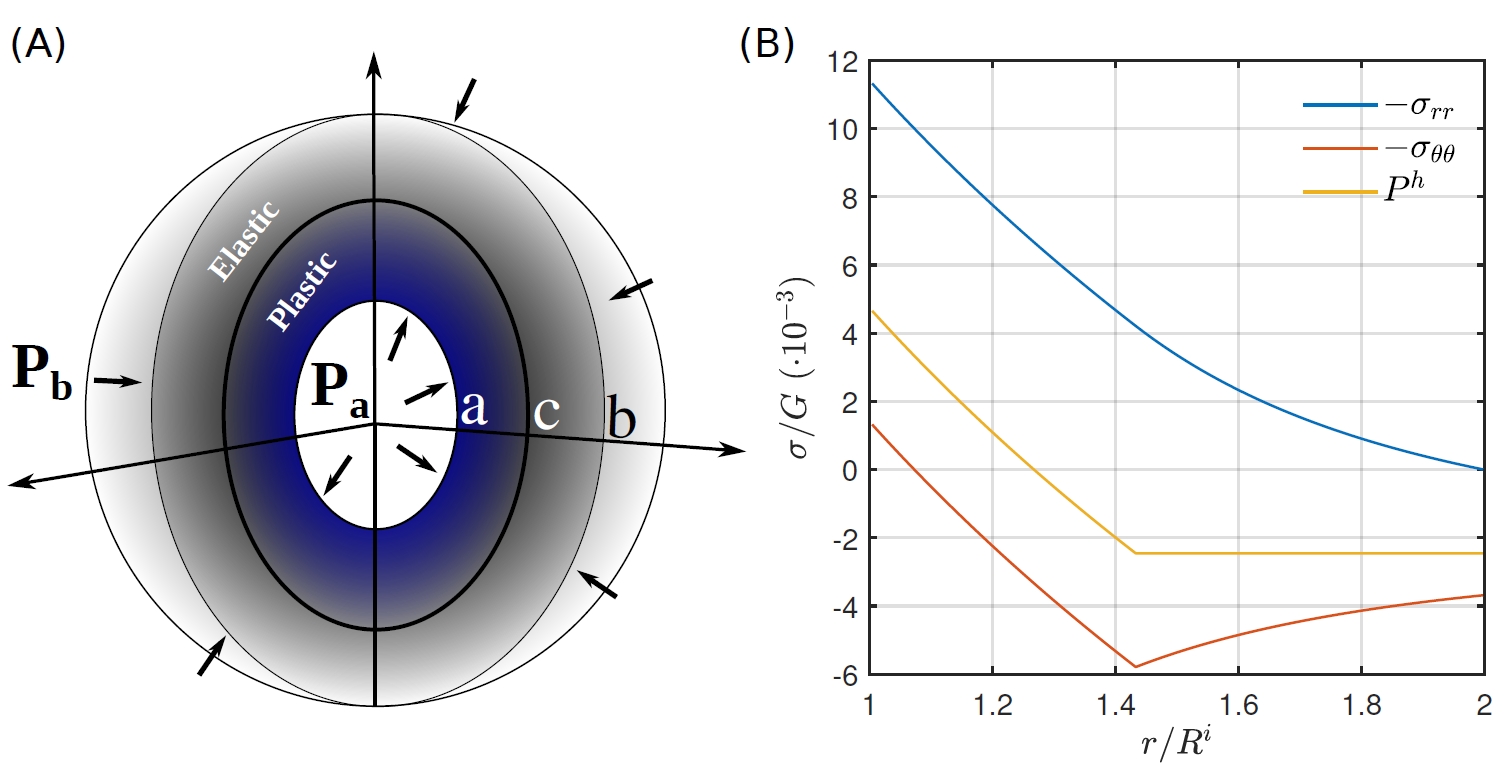
A: Schematic representation of the elasto-plastic zone that develops at a host-inclusion interface. B: stress distribution around a pressurized mineral inclusion (see publications [21, 31, 32] for more details).
Thermodynamic equilibrium and stress evolution of metamorphic rocks
[in collaboration with Dr. M. Mazzucchelli (Uni. Lausanne), Prof. Dr. S. Schmalholz (Uni. Lausanne) & Prof. Dr. T. Speck (Uni. Stuttgart)]
The development of stresses in minerals affects their energy. As a result, the stability of metamorphic mineral assemblages does not depend on a simple “pressure” value and more complex theories are needed. In this research direction, we investigate the thermodynamic equilibrium of stressed rocks by means of:
i) deformation experiments corroborated by thermomechanical simulations
ii) hydro-chemo-mechanical modelling (multiphase flow equations)
iii) classical molecular dynamics simulations
Our results can be used to create predictive models that can account for the effects of deformation on mineral reactions (see publications [17, 22, 30, 35, 38] for more details).
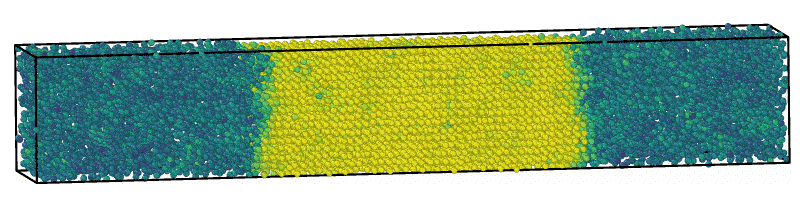
Example of a molecular dynamics simulation of the coexistence of a stressed solid with a melt of the same composition (see publication [38] for more details)
Modelling of CHROMium Enrichment in the mantle and the crust (CHROME)
[in collaboration with Prof. Dr. Boris Kaus (Uni. Mainz), Prof. Dr. R. Botcharnikov (Uni. Mainz), Dr. N. Riel (Uni. Mainz), Dr. S. Buhre (Uni. Mainz), Prof. Dr. D. Kostopoulos (Uni. Athens) and Prof. Dr. P. Pomonis (Uni. Athens) – funded by DFG]
The CHROME project aims to create models for understanding the formation of chromite deposits in ophiolites, focusing on the thermodynamic behavior of multiphase flows. It investigates how chromium behaves during the melting and crystallization processes, noting that despite Cr’s compatibility, its concentration in melts is not sufficient alone to form chromite deposits, suggesting additional enrichment processes are necessary. The project plans to model the dynamics of melt transport and reactive flows, incorporating various possible enrichment mechanisms, to test hypotheses on chromite formation. Experiments on peridotite rocks containing chromium and multiphase, reactive flow modeling are planned to complement each other, offering insights into ore formation processes. This integrated approach aims to refine our understanding of ore formation, taking into account both experimental results and observations from natural deposits.
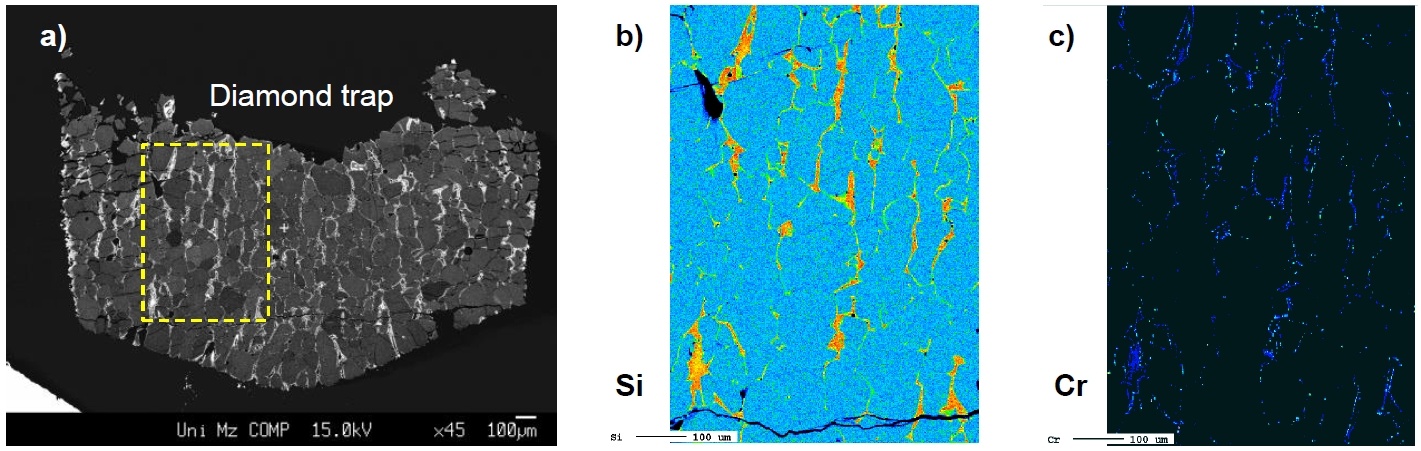
Experimental result from natural peridotites (Back-Scattered-Electron Image). (a) The bright areas correspond to interconnected channels of Cr-rich silicate melt on its way to the diamond melt trap (at the top side of the sample). (b) and (c) Elemental maps for Si and Cr, respectively, taken in the area outlined by yellow dashed line in (a).
Viscous EffeCTs On Raman-based elasto-thermobarometry (VECTOR): a field, mechanical and diffusion study of mineral inclusions entrapped under a wide range of metamorphic conditions
[in collaboration with Prof. Dr. J. Szczepański (Uni. Wroclaw) and Prof. Dr. M. Dąbrowski (Polish Geol. Inst.) – funded by the DFG and the National Science Centre (PL)]
Recent advancements like Raman spectroscopy-based elasto-thermobarometry offers a new way to estimate the re-equilibration conditions in a rock’s history. However, the high-temperature irreversible deformation (viscous creep) has been identified to compromise the integrity of minerals’ elastic properties, affecting their ability to retain residual pressure in inclusions. The project aims to develop a new method to quantify the impact of viscous creep on mineral inclusions’ capacity to hold residual pressure, addressing a gap in systematic studies on this issue. Through integrating field data, analytical measurements, and numerical modeling of re-equilibration processes at the mineral level, this project seeks to quantitatively assess the effects of viscous creep and refine the accuracy of determining metamorphic conditions using Raman thermobarometry.
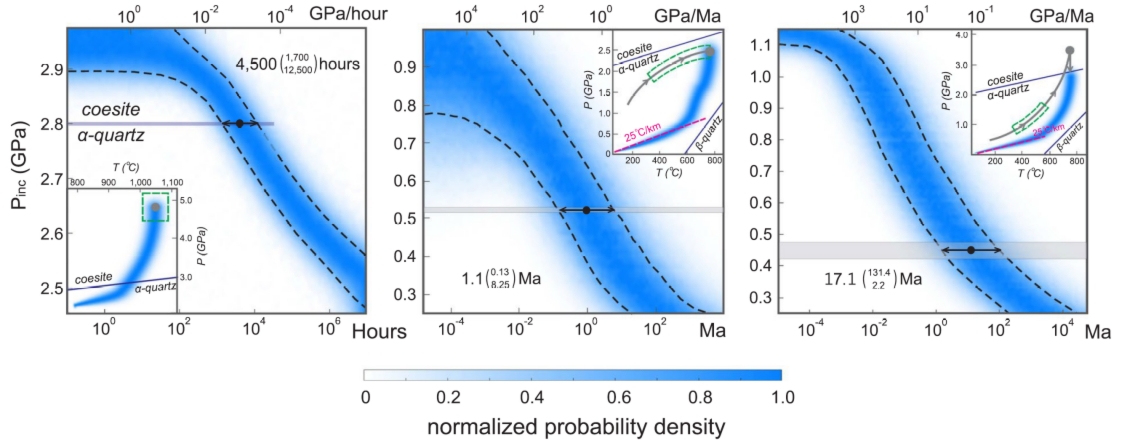
Timescale predictions for the exhumation of host-inclusion systems. The predictions are based on the numerical models of temperature-dependent viscoelastic relaxation (see publication [13] for more details).
A combination of petrological and joint chemical-mechanical inversion approaches to unravel deep geodynamic processes
[in collaboration with Dr. I. Ibragimov (Uni. Mainz) – funded by the DFG]
Metamorphism involves the recrystallization of rocks under high temperatures, subjecting them to various pressure and temperature conditions that lead to chemical and mechanical changes in their structure. As metamorphic rocks are brought to the surface, they often retain “frozen” evidence of these processes due to the rapid cooling they undergo, preserving high-temperature traces like chemically zoned crystals and stresses within mineral inclusions. Recent advancements in diffusion modeling and Raman elastic barometry have enhanced our understanding of these changes in natural rocks. This study aims to develop inverse models that could jointly infer the mechanical and chemical relaxation of metamorphic textures. Such models can be corroborated using geodynamic models that predict the P-T evolution of rocks. Focusing on metamorphic rocks, the approach seeks to quantify the slow, often imperceptible metamorphic processes that occur under the Earth’s surface (see for example publication numbers [39] and [40] for more details).
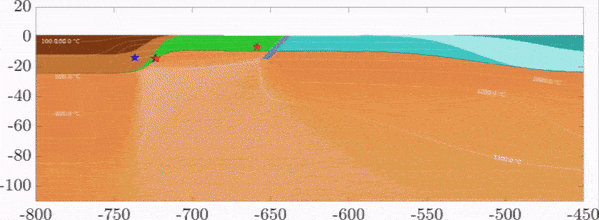
Geodynamic simulation of lithospheric convergence and continental subduction. The different rock units move through space and experience a variety of conditions. Thermally-activated processes such as solid-state mineral diffusion and viscous relaxation (creep) will occur in the deforming metamorphic rocks depending on the different paths taken by the rocks. The forward simulation of such processes will lead to the better understanding of the integrated geological data.